Understanding CO2 Sensors for 10,000 PPM Applications
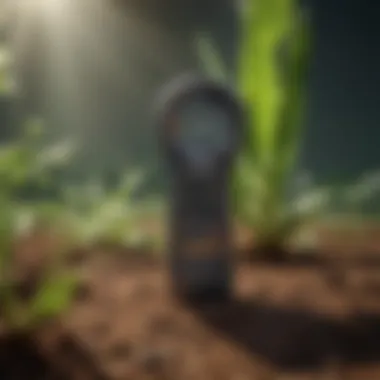
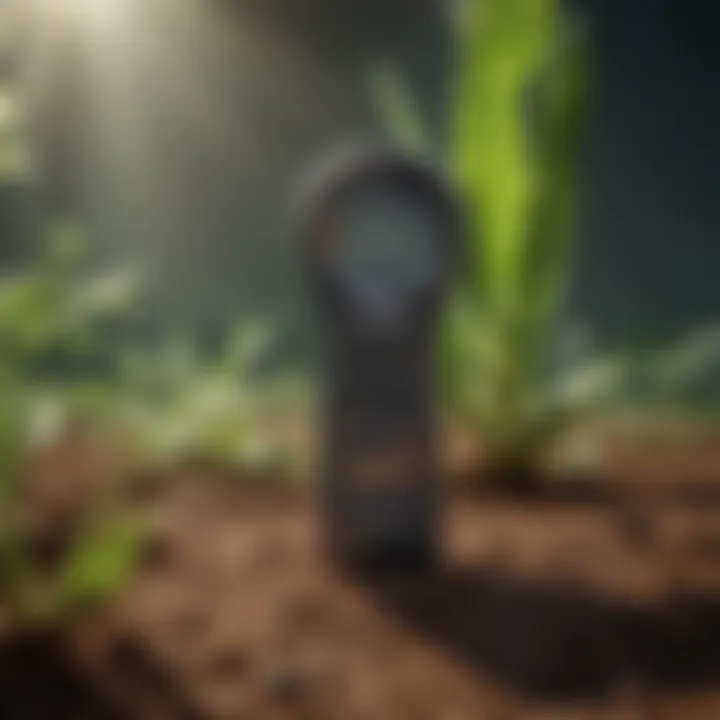
Intro
Carbon dioxide (CO2) sensors play a pivotal role in various industries. They are particularly crucial for applications that require monitoring CO2 levels up to 10,000 parts per million (PPM). Understanding these sensors is essential for effective decision-making in sectors such as agriculture, environmental science, and industrial processes. This section offers insight into their functionality and importance.
Overview of the Topic
Definition and Importance
CO2 sensors are instruments designed to measure the concentration of carbon dioxide in the environment. In applications that involve high levels of CO2, precise measurements are crucial. High CO2 concentrations can affect plant growth and productivity in agriculture. Likewise, in industrial scenarios, it may impact safety and operational efficiency.
Monitoring CO2 levels effectively promotes sustainable practices. It allows stakeholders to identify problematic conditions early, thus minimizing risks and optimizing processes. For farmers, understanding CO2 levels can significantly improve crop yield and quality.
Current Trends
The market for CO2 sensors has evolved. Advances in technology have resulted in enhanced precision and reliability. More industries are recognizing the importance of real-time data for operational efficiency. Moreover, there is an increasing demand for wireless sensors, which allow for easier integration within existing systems.
New regulations are emerging, emphasizing the need for accurate CO2 monitoring. These factors contribute to a growing interest in 10,000 PPM CO2 sensors across various applications.
Key Techniques and Practices
Step-by-Step Guide
- Identify Requirements: Determine the specific CO2 levels that need monitoring based on your application.
- Select Appropriate Sensor: Choose from different types of sensors like non-dispersive infrared (NDIR) sensors, which offer high accuracy.
- Calibration: Regularly calibrate your sensors to maintain accuracy. Calibration standards often depend on the specific application.
- Data Collection: Gather CO2 level data at regular intervals. Set parameters for data logging and management.
- Analysis: Evaluate data to make informed decisions about the conditions of your crops or industrial environment.
Tools and Equipment Needed
- CO2 sensors (e.g., Aqualung Sensor or Vaisala GMP252)
- Calibration gas (specific to the sensor used)
- Data logging software (such as Excel or specialized apps)
- Cleaning supplies for sensor maintenance
Challenges and Solutions
Common Obstacles
There are challenges when working with CO2 sensors. For instance, sensor drift can affect accuracy over time. Additionally, environmental factors can interfere with readings, making maintenance essential.
Innovative Solutions
To counter these challenges, regular calibration is necessary. Implementing a maintenance schedule helps ensure accuracy. Furthermore, using advanced sensors with self-calibrating features can reduce the workload associated with manual calibrations.
Intro to CO2 Sensors
Understanding CO2 sensors is crucial in a world where the balance of gases impacts environmental health and agricultural productivity. These sensors are essential for monitoring carbon dioxide levels, especially when considering applications that reach up to 10,000 parts per million (PPM). Such precision helps various sectors, from farming to industrial processes, by ensuring optimal conditions for growth and efficiency. As global challenges increase, the role of CO2 monitoring becomes ever more significant.
Definition and Functionality
CO2 sensors are devices designed to measure the concentration of carbon dioxide in the air. They operate through different mechanisms depending on their type, such as non-dispersive infrared absorption, chemical reactions, or photoacoustic effects. Each method has its advantages in terms of accuracy, response time, and suitable application areas.
For instance, non-dispersive infrared sensors are commonly used due to their reliability and ability to function over extended periods without significant drift. On the other hand, chemical sensors, while often less expensive, can be affected by environmental conditions, which may require more frequent calibration.
The functionality of these sensors extends beyond simple measurement. They not only help track current CO2 levels but also provide critical data for systems that rely on controlled environments, such as in agriculture and greenhouse management. In this way, they function as both monitoring tools and control systems, sometimes triggering alarms or adjustments in response to changing CO2 concentrations.
Importance of CO2 Monitoring
The monitoring of CO2 is not just a technical requirement; it is vital for maintaining healthy environments in various sectors. In agriculture, for example, elevated CO2 levels can enhance photosynthesis, thus improving plant growth and crop yields. Conversely, too much CO2 can be detrimental, leading to reduced yield or crop quality.
Proper CO2 monitoring can lead to informed agricultural practices. Farmers can optimize conditions for plants, ensuring they receive the ideal balance of gases for maximum yield. Moreover, in industrial settings, monitoring CO2 emissions helps companies comply with environmental regulations, reducing their carbon footprint. This aligns with broader efforts to combat climate change.
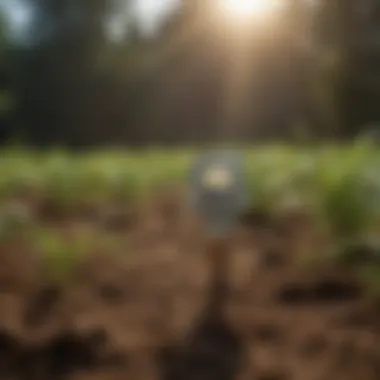
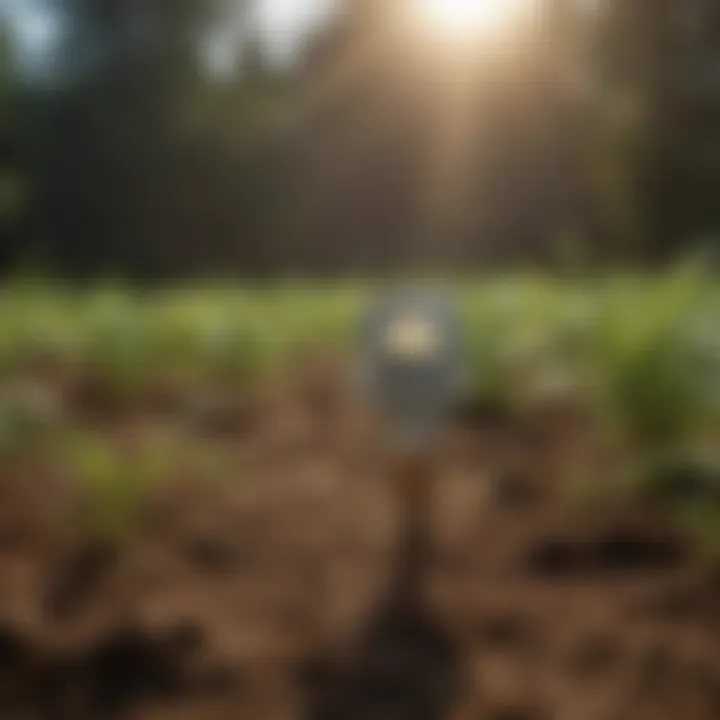
"Effective CO2 monitoring is fundamental not just for compliance, but for sustainable practices that benefit both the economy and the environment."
In essence, understanding and implementing CO2 sensors is pivotal not only for immediate gains in productivity but also for long-term sustainability. The importance of CO2 monitoring, therefore, extends beyond measurements; it influences decision-making processes and fosters better practices across sectors.
Understanding PPM in CO2 Measurements
Understanding Parts Per Million (PPM) is essential in comprehending how CO2 sensors operate within various sectors, particularly those requiring precision in measurements. In the context of CO2 sensor technology, PPM denotes the concentration of carbon dioxide within air or other gases. This is a pivotal metric, especially when considering applications that demand monitoring of high concentrations, such as up to 10,000 PPM, as seen in specific industrial and agricultural scenarios.
What is Parts Per Million?
Parts Per Million is a unit of measurement that signifies the ratio of one part of a substance to one million parts of a whole. In atmospheric science and environmental studies, it allows for the quantification of trace gases, such as carbon dioxide, in the atmosphere.
To put PPM into perspective, if one considers a sample of air with 1,000,000 molecules and 400 of those are CO2, the concentration would be reported as 400 PPM. In this way, PPM provides a clear and authoritative description of gas concentrations, making it fundamental in settings where maintaining specific gas levels is critical.
These measurements can influence regulations and health guidelines, as high CO2 levels might indicate poor air quality or potential hazards in confined environments.
Relevance of , PPM
The relevance of monitoring CO2 concentrations up to 10,000 PPM is significant across multiple sectors. In agriculture, for instance, controlled environments such as greenhouses can benefit from higher levels of CO2, stimulating photosynthesis and thus enhancing plant growth. However, levels approaching 10,000 PPM can pose risks to human health if not managed properly, underscoring the importance of accurate monitoring.
In industrial applications, these sensors help ensure that processes are operating within safe limits. It is not merely a matter of tracking concentration for the sake of data; it dictates operational standards and health regulations. Hence, understanding and utilizing CO2 sensors in the context of high PPM levels demands a thorough grasp of both the technology and its implications.
CO2 sensors equipped to detect levels approaching 10,000 PPM are crucial for achieving sustainable practices that balance productivity with safety. The capacity to monitor and adjust CO2 levels allows industries to optimize resource use, ensuring that farming and manufacturing processes do not jeopardize health or environmental quality.
Types of CO2 Sensors
Understanding the types of CO2 sensors is crucial for anyone involved in applications requiring precise atmospheric monitoring, especially in environments that experience CO2 levels up to 10,000 parts per million (PPM). Each sensor type has unique characteristics, advantages, and limitations, making them suitable for specific scenarios. This section will explore the three primary categories of CO2 sensors: Non-Dispersive Infrared Sensors, Chemical Sensors, and Photoacoustic Sensors.
Non-Dispersive Infrared Sensors
Non-Dispersive Infrared (NDIR) sensors are among the most common technologies used for measuring CO2 levels. They operate on the principle that CO2 molecules absorb specific wavelengths of infrared light. An infrared light source emits light through a detection chamber containing the air sample. A detector measures the amount of light that passes through the chamber. The more CO2 present, the less light reaches the detector, allowing for accurate concentration measurements.
Benefits of NDIR Sensors
- Accuracy: NDIR sensors provide high accuracy, which is essential for applications like greenhouse monitoring.
- Stable Readings: These sensors have the capacity for stable long-term readings with minimal drift.
- Wide Range: They can measure a broad range of CO2 levels, suitable for scenarios where concentrations may reach up to 10,000 PPM.
Chemical Sensors
Chemical sensors are another option for detecting CO2. They utilize a chemical reaction to measure gas concentrations. A chemical compound in the sensor reacts with CO2, producing a detectable change, often in the form of a voltage or current. These sensors can be less expensive than NDIR sensors but come with their own set of considerations.
Pros and Cons of Chemical Sensors
- Cost-Effective: Chemical sensors are generally cheaper, making them attractive for smaller operations or budget-conscious projects.
- Limited Range: They may not accurately measure high concentrations of CO2, which can be a significant drawback in environments reaching 10,000 PPM.
- Sensitivity to Other Gases: Chemical sensors may be influenced by other gases, potentially leading to inaccurate readings.
Photoacoustic Sensors
Photoacoustic sensors work on the principle of sound wave production in a gas when it absorbs light. When CO2 absorbs light energy, it causes a slight but detectable change in pressure within the sensor, creating sound waves. These sensors can provide accurate measurements and are becoming increasingly popular in various applications.
Advantages of Photoacoustic Sensors
- High Sensitivity: They can detect very low concentrations of CO2, making them suitable for monitoring in sensitive environments.
- Rapid Response: These sensors typically offer quick response times, essential for real-time monitoring.
- Resistance to Interference: Photoacoustic sensors tend to be less affected by other gases, providing more reliable data in mixed gas environments.
Calibration of CO2 Sensors
Calibration of CO2 sensors is a critical process that determines the accuracy and reliability of measurements in environments where levels can reach up to 10,000 PPM. The precision of CO2 sensors has direct implications on various applications, particularly in agriculture, environmental monitoring, and industrial settings. Knowing how to properly calibrate these sensors not only enhances data accuracy but also ensures compliance with safety regulations and operational guidelines.
Importance of Calibration
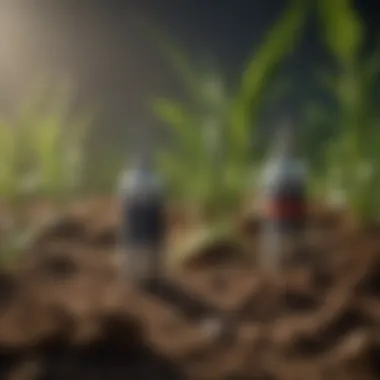
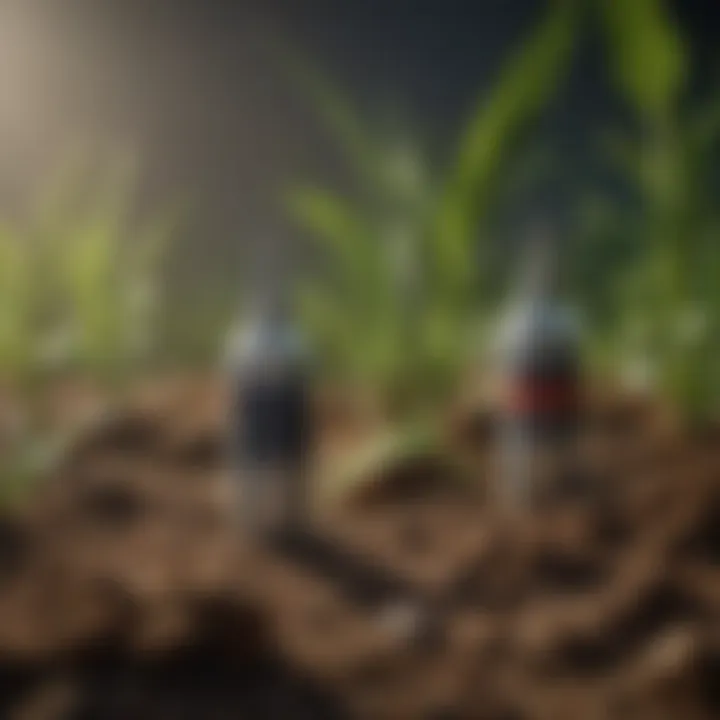
Calibration plays a vital role in ensuring that CO2 sensors operate within their specified accuracy range. Sensor drift can occur over time due to various environmental factors. This drift can lead to erroneous readings that misguide decision-making processes.
Proper calibration prevents potential losses in agricultural yields or adverse effects on industrial processes. The significance of accurate CO2 measurements cannot be overstated; smooth operations rely heavily on trustworthy data. Additionally, through precise calibration, operators can identify sensor malfunctions early, reducing downtime associated with unexpected sensor failures.
Methods of Calibration
Various methods exist for calibrating CO2 sensors, and the choice may depend on the specific application or sensor type. Common methods include:
- Zero Calibration: This method establishes a baseline signal that represents a zero CO2 reading. It is essential for sensors that measure in environments where CO2 is minimal.
- Span Calibration: This method involves exposing the sensor to a known concentration of CO2. It adjusts the sensor's output to correlate with the concentration, ensuring that the readings maintain accuracy under operational conditions.
- Multi-point Calibration: Sometimes, sensors are calibrated at several points along the measurement range. This provides a comprehensive understanding of sensor accuracy across different levels of CO2 concentration.
While calibration can be done manually, some sensors feature automatic calibration processes, streamlining the regular maintenance of equipment.
Frequency of Calibration
The frequency of calibration will depend on several factors, including the specific application, sensor type, and environmental conditions. It is advisable for users to implement a regular calibration schedule, typically every six months to yearly, although specific conditions may require more frequent adjustments.
Factors influencing calibration frequency include:
- Environmental Variability: Sensors exposed to extreme temperature fluctuations or humidity changes may require more frequent calibration.
- Usage Intensity: If a sensor is frequently used or subjected to high CO2 levels, it may drift more noticeably and often need recalibration.
- Manufacturer Recommendations: Always consult the manufacturer's guidelines for specific recommendations related to calibration intervals.
Regular calibration is essential to maintaining the reliability of CO2 sensors, especially in agricultural environments where precise data informs critical farming decisions.
Applications of High-Range CO2 Sensors
High-range CO2 sensors play an essential role in various sectors. They provide vital data that supports environmental, agricultural, and industrial advancements. Understanding their applications helps realize the broader implications of CO2 measurement. These sensors can influence how resources are managed, how crops grow, and how industries operate. The accurate data from these sensors aids in decision making and planning.
Agricultural Practices
In agriculture, CO2 levels significantly affect plant photosynthesis and growth. By monitoring CO2 concentrations, farmers can optimize conditions for crop production. High-range CO2 sensors can be instrumental in developing strategies that lead to higher yields.
- Enhanced Growth: Consistent monitoring can help maintain ideal CO2 levels, ensuring plants receive enough for optimal growth.
- Reduced Waste: Farmers can minimize resource waste by only using fertilizers when necessary, guided by CO2 readings.
- Improved Sustainability: Utilizing these sensors promotes sustainable practices. They allow farmers to balance their outputs with environmental needs.
Greenhouse Management
Greenhouses thrive on maintaining specific environmental conditions. Integrating CO2 sensors in these structures provides real-time data on airflow and gas concentrations. This leads to improved control of the growing environment.
- Climate Control: By knowing the CO2 levels, managers can adjust ventilation systems. This ensures healthy growing conditions without excess gas accumulation.
- Crop Health Monitoring: Sensors can help identify problems earlier. If CO2 levels are not where they should be, it may signal a larger issue, such as poor ventilation or plant decay.
- Efficiency: With precise data, energy consumption for heating or cooling can be minimized. This leads to cost savings and a lower carbon footprint.
Industrial Processes
Industries that rely on precise environmental parameters can benefit significantly from high-range CO2 sensors. Many manufacturing processes produce CO2 as a byproduct and monitoring this is crucial for safety and efficiency.
- Safety Compliance: Keeping CO2 levels within safe limits helps reduce occupational hazards. Workers are less likely to be exposed to dangerous concentrations.
- Process Optimization: Real-time data enables facilities to optimize processes. This can increase production efficiency and reduce costs.
- Regulatory Compliance: Many industries face strict regulations regarding emissions. High-range CO2 sensors contribute to adherence to these regulations, avoiding potential fines and shutdowns.
Accurate CO2 measurements are crucial in modern practices across sectors. They not only enhance productivity but also foster sustainable methods.
Benefits of Implementing CO2 Sensors
Implementing CO2 sensors that can detect levels up to 10,000 PPM greatly influences multiple sectors, particularly in agriculture, environmental monitoring, and industrial processes. The utilization of these advanced sensors allows practitioners to optimize their operations and improve productivity while ensuring environmental sustainability. Elevated levels of carbon dioxide can significantly impact plant health, air quality, and operational efficiency. Therefore, understanding the benefits can guide stakeholders in making informed decisions about their use.
Enhanced Environmental Monitoring
Enhanced environmental monitoring is a crucial advantage of CO2 sensors. These devices provide real-time data on CO2 concentration levels, enabling better air quality management. With accurate readings, stakeholders can implement corrective actions promptly. This monitoring is particularly important in areas prone to CO2 buildup, such as greenhouses or confined spaces.
Effective monitoring helps to:
- Protect against harmful concentrations of CO2 that may negatively affect human health, plants, and animals.
- Comply with environmental regulations that require monitoring and control of greenhouse gases.
- Generate vital data for research in climate change and its effects on ecosystems.
Accurate CO2 measurements can lead to better decision-making, ultimately supporting sustainable practices.
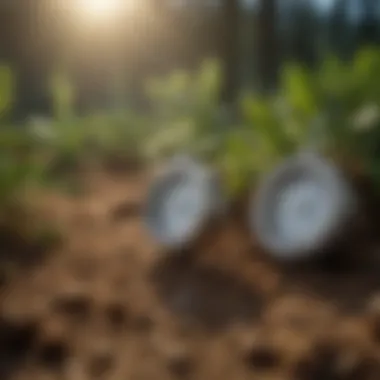
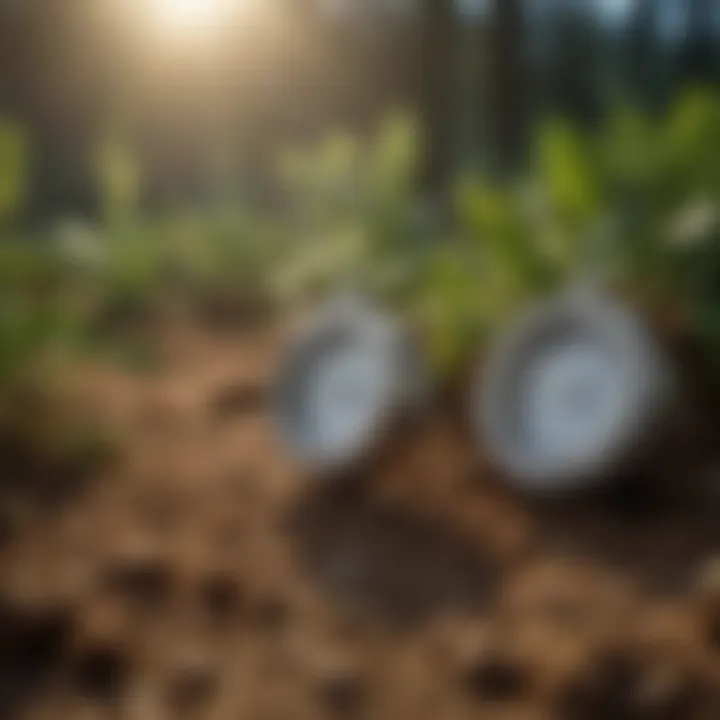
Improved Crop Yields
Improved crop yields are another critical benefit directly related to the implementation of high-range CO2 sensors. By understanding and monitoring the exact levels of carbon dioxide in agricultural settings, farmers can make data-driven decisions to optimize conditions for plant growth. High CO2 levels can enhance the photosynthesis process, which can in turn lead to increased crop productivity. Specific benefits include:
- Tailored fertilization strategies that align with carbon levels, improving nutrient uptake by crops.
- Prevention of adverse conditions that may arise from excessive CO2 concentrations, such as reduced photoperiod and plant stress.
- Increased awareness of CO2 levels affecting different crop varieties, allowing for selection of optimal growth conditions.
Optimization of Resource Use
The optimization of resource use is a significant advantage that comes with the deployment of CO2 sensors. With precise data at hand, farms and industries can manage their resources more effectively, resulting in reduced waste and cost savings. This can manifest in several ways:
- Efficient use of energy in heating and cooling systems, which can be correlated with CO2 levels.
- Reduced water usage by adjusting irrigation schedules based on environmental data.
- Better planning for inputs like fertilizers and pesticides, which contributes to lowering overall production costs.
With these advantages in mind, embracing CO2 sensors represents a clear step towards more responsible and efficient practices in agriculture and beyond.
Limitations and Challenges
Understanding the limitations and challenges associated with CO2 sensors is essential for effective usage, particularly in high-range applications. Despite their advantages, these sensors come with specific drawbacks that users need to consider. Addressing these challenges is vital to maximize their efficiency and accuracy in real-world applications, especially in agriculture and industry.
Sensor Drift
Sensor drift refers to the gradual shift in the sensor's output signal over time, which can lead to inaccuracies in CO2 measurement. This phenomenon can happen due to various factors, such as aging components, changes in environmental conditions, or long-term exposure to high concentrations of CO2. Regular calibration is necessary to mitigate the effects of drift. Otherwise, the data collected could mislead decision-makers relying on accurate measurements to optimize their processes. For instance, a farmer might misjudge the CO2 levels in a greenhouse, leading to inefficient resource utilization and reduced crop yields.
Interference from Other Gases
CO2 sensors can sometimes detect other gases in addition to carbon dioxide. This interference can distort the readings, potentially resulting in erroneous conclusions. For example, if methane or volatile organic compounds are present, the sensor may register higher CO2 levels than actual. This is particularly problematic in environments with multiple gases, such as in industrial settings. Selecting the right type of sensor is crucial to minimize interference. Non-dispersive infrared sensors are generally less prone to this issue, but still might not be completely immune. Being aware of these interferences is critical for proper analysis and results.
Cost Considerations
The cost of CO2 sensors can vary significantly based on type, accuracy, and application range. While investing in high-quality sensors may provide better performance, budget constraints often influence decisions, especially in agricultural sectors. Users must weigh the initial costs against potential future savings from improved efficiency and productivity. Furthermore, maintenance and calibration can add to the overall investment in CO2 monitoring equipment. Making a choice between cheaper sensors and more reliable options is a challenge that requires careful evaluation. Determining the total cost of ownership—including maintenance and calibration—helps in making an informed decision that aligns with long-term goals.
Future Trends in CO2 Sensing Technology
The domain of CO2 sensing technology is not static; it continues to evolve rapidly. These advancements on multiple fronts are pivotal, especially for applications reaching up to 10,000 PPM. Understanding these trends can empower agriculture farmers and enthusiasts to utilize CO2 sensors more effectively.
Advancements in Sensor Durability
Sensor durability is critical for reliable monitoring. The harsh environments often found in agricultural settings can lead to sensor degradation. Technological advancements now focus on materials and design that enhance resilience. For instance, sensors with improved casing materials withstand moisture and temperature fluctuations. Additionally, manufacturers are employing advanced coating techniques that resist dust and corrosive elements. This longevity translates into lower maintenance costs and less frequent replacements. The ability to maintain accuracy over extended periods is especially vital in environments with varying CO2 levels.
Integration with IoT
The integration of CO2 sensors with Internet of Things (IoT) technology marks a significant leap forward. Farmers can now utilize real-time data to adapt their practices. Smart sensors transmit information wirelessly, allowing for immediate analysis. This connectivity can alert users to critical changes in CO2 levels without manual checks. Moreover, integrating with platforms like cloud computing enhances data storage and accessibility. Analyzing historical data to identify trends helps in making informed decisions. This technology not only enhances productivity but also contributes to sustainable farming practices.
Data Analytics in CO2 Monitoring
Data analytics plays a crucial role in interpreting the information gathered by CO2 sensors. As agriculture becomes increasingly data-driven, the ability to extract valuable insights from CO2 measurements becomes important. Advanced algorithms can analyze patterns, correlating CO2 levels with crop health and environmental factors. This capability supports targeted interventions, optimizing resources such as fertilizers and water. The potential to fine-tune growing conditions based on data can dramatically enhance crop yields. Thus, having sophisticated analytics tools alongside reliable sensors reinforces the decision-making process for farmers.
"The future of agriculture hinges on our capacity to adapt and monitor our environment in real-time."
The End
The conclusion section of this article serves several key purpose. It encapsulates the primary insights about CO2 sensors designed for high concentration applications, particularly those capable of invasive detection up to 10,000 PPM. The significance of reliable data from these sensors cannot be overstated, especially in environments where precise carbon dioxide monitoring is critical for both productivity and safety.
Summary of Insights
In this article, we covered various aspects of CO2 sensors, highlighting their functionality and types such as Non-Dispersive Infrared Sensors, Chemical Sensors, and Photoacoustic Sensors. We examined how calibration aids in maintaining accuracy and the diverse applications in agriculture, industrial processes, and environmental management. The necessity for ongoing monitoring ensures that stakeholders in these sectors make informed decisions. The benefits of implementing CO2 sensors extend beyond simply regulating gas levels; they include enhanced yields in farming and optimized resource use in industrial settings.
Final Thoughts on CO2 Measurement Practices
It is clear that CO2 measurement practices evolve to meet the challenges of today's agricultural and industrial demands. As future technologies integrate seamlessly with advanced sensors, the role of high-precision CO2 monitoring will become increasingly pivotal. Engaging in best practices, from calibration to employing the right type of sensor for specific applications, becomes essential in maximizing advantages and minimizing risks.
In closing, the impact of accurate CO2 monitoring is profound. Embracing these technologies not only addresses immediate operational concerns but also aligns with larger sustainability goals that benefit both industries and the environment alike. Investing in such advancements represents a forward-thinking approach that can yield considerable returns, both economically and ecologically.
"Effective CO2 monitoring is not just about measurement; it's about understanding the very dynamics of our environment and making informed decisions based on real-time data."